Verification of Quantum Electrodynamics Using Exotic Atoms
An international collaboration of researchers, including the Kavli Institute for the Physics and Mathematics of the Universe (Kavli IPMU) has succeeded in a proof-of-principle experiment to verify strong-field quantum electrodynamics with exotic atoms, by performing high-precision measurements of the energy spectrum of muonic characteristic X-rays emitted from muonic atoms using a state-of-the-art X-ray detector, reports a new study in Physical Review Letters.
The group’s results are a significant step toward verifying fundamental physical laws under strong electric fields, which humankind has not yet been able to create artificially. The highly efficient and accurate X-ray energy determination method using state-of-the-art quantum technology demonstrated in this research is expected to be applied to various research fields, such as non-destructive elemental analysis methods using muonic atoms.
It has always been a dream of scientists to discover physical laws. They have been found or proposed to explain observed phenomena that cannot be understood by existing theories. In many cases, the discovery of new physics requires the development of new experimental techniques and improved accuracy of measurements. The most precisely tested theory of physical laws is Quantum ElectroDynamics (QED), which describes the microscopic interactions between charged particles and light. Scientists are constantly pushing the limits of how far QED accurately describes our physical reality.
In this paper, the collaboration injected a low-velocity negative muon beam from the J-PARC facility into neon gas, and the energy of characteristic X-rays emitted from the resulting muonic neon (Ne) atoms was precisely measured using a superconducting Transition-Edge Sensor (TES) detector. By taking full advantage of the excellent energy resolution of the TES detector, the energy of the muonic characteristic X-rays was determined with an absolute uncertainty of less than 1/10,000, and contributions from vacuum polarization in strong-field quantum electrodynamics were successfully verified with a high precision of 5.8 %.
The TES detector was originally developed for space X-ray observation. Takahashi’s current project at Kavli IPMU has been to carry out unprecedented cross-disciplinary research using this detector. His team includes Kavli IPMU Project Assistant Professor Shin’ichiro Takeda, Project Researcher Miho Katsuragawa, and at-the-tune graduate student Kairi Mine, who took part in the muon experiments.
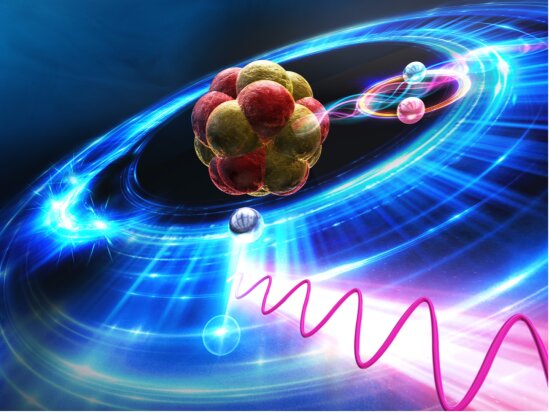
The collaboration’s demonstration of the experimental technique using muonic atoms is expected to lead to a great leap forward in the study of QED verification under strong electric fields.
Details of the study are published in the journal Physical Review Letters.
The effects of QED are more pronounced in environments with strong electric fields, but theoretical calculations become more difficult in this case. Therefore, a strong electric field environment is very important for QED verification. For many years, experiments using highly charged ions (HCIs), which are atoms stripped of multiple electrons, have been conducted as an approach to realize a strong electric field environment.
The electric field felt by the bound electrons in HCIs becomes stronger as the atomic number becomes larger, and the shielding effect is suppressed by the stripping of many electrons. HCI research using large accelerators is still vigorously pursued. However, even for HCIs with large atomic numbers, the effect of the finite size of the nucleus cannot be ignored. It has been pointed out that this effect is not precisely known, and thus the accuracy of QED verification, which compares experimental results with theory, is greatly compromised.
To verify QED under strong electric fields in a different way than with HCIs, international research groups have focused on “exotic atoms,” in which a negatively charged particle is bound to the nucleus instead of the electron. Among the variety of exotic atoms, muonic atoms are composed of negative muons (elementary particles about 200 times heavier than electrons) and nuclei.
Negative muons can nowadays be extracted as beams from large accelerators. Muonic atoms are characterized by the extremely close proximity of the negative muon to the nucleus, with the orbital radius of a bound muon being approximately 1/200th that of a bound electron.
As a result, the electric field felt by the muon is about 40,000 times stronger than the electric field felt by a bound electron of the same quantum level in an HCI, resulting in a huge QED effect. In addition, by using negative muons, which occupy high angular momentum quantum levels with small overlap with the nucleus, it is possible to conduct experiments in which the effect of the finite size of the nucleus is largely suppressed. By precisely measuring the energy of muonic characteristic X-rays emitted when muonic atoms are deexcited from a specific level to lower levels, QED can be verified under a strong electric field (Figure 1).
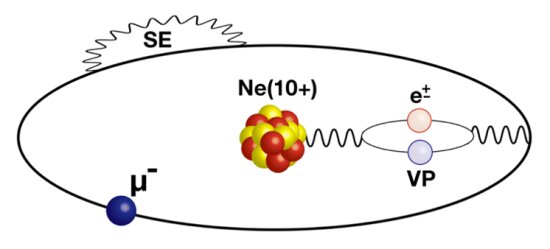
Thus, muonic atoms are a promising experimental target for strong-field QED verification. However, there are several problems to overcome. The largest is that a number of muonic atoms must be prepared in an isolated environment. The presence of atoms or molecules in the vicinity of the muonic atoms may cause rapid electron transfer and changes the energy of the muonic characteristic X-rays. The solution is to use dilute gas targets with a small number density (low pressure), but the number of produced muonic atoms and the resulting intensity of muonic characteristic X-rays are reduced.
The international research group conducted experiments at the Japan Proton Accelerator Research Complex (J-PARC) in Tokai-mura, Ibaraki, where the world’s most intense low-velocity muon beam is available. In order to determine the energy with sufficient accuracy even with low-intensity muonic characteristic X-rays, the experiment was conducted with a superconducting transition edge sensor (TES) microcalorimeter, which is a highly efficient and high-resolution X-ray detector.
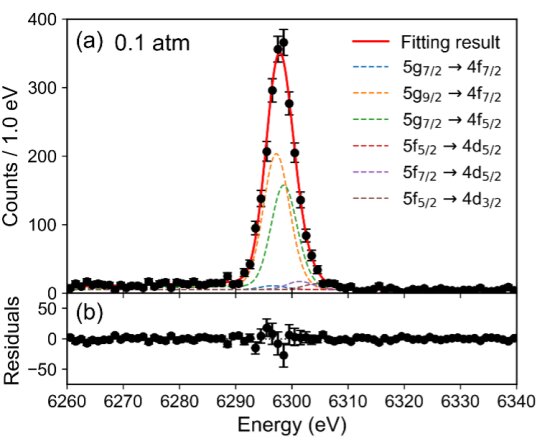
Using rare gas neon (10Ne) atoms as the target, they have achieved an energy resolution that is one order of magnitude higher than that of conventional semiconductor detectors (FWHM [11]: 5.2 eV) under dilute conditions of 0.1 atm and successfully measured the muonic characteristic X-rays (Figure 2). The peaks shown are mainly due to the overlap of muonic characteristic X-rays from six different transitions, and the energy of the muonic characteristic X-rays was determined to a high accuracy of 0.002% by analyzing contributions from each of them.
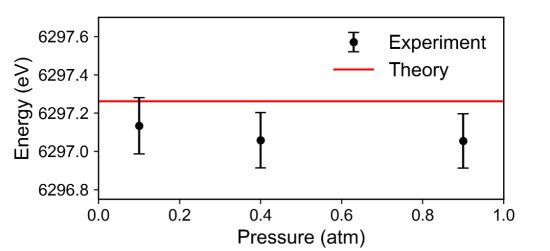
They repeated the measurements while changing the pressure of the neon gas target (Figure 3) and confirmed that the energy of the muonic X-rays is constant within experimental error regardless of the pressure of the neon gas target.
Thus, it can be concluded that the muonic neon atoms were in an isolated environment. They compared the latest theoretical calculations with the experimental results and confirmed that they agreed within the experimental error. The researchers succeeded in verifying the effect of vacuum polarization under a strong electric field with an extremely high accuracy of 5.8%. This is comparable to the accuracy of strong-field QED using the multiply charged uranium ion U91+, which is the most accurate observation to date.
This article is republished from PhysORG under a Creative Commons license. Read the original article.
Do not forget to share your opinion with us to provide you with the best posts !
0 Comments