Understanding the Illumination Mechanism as a Black Hole Tears Apart a Star
A novel model monitors the remnants of a celestial body orbiting a gravitational singularity.
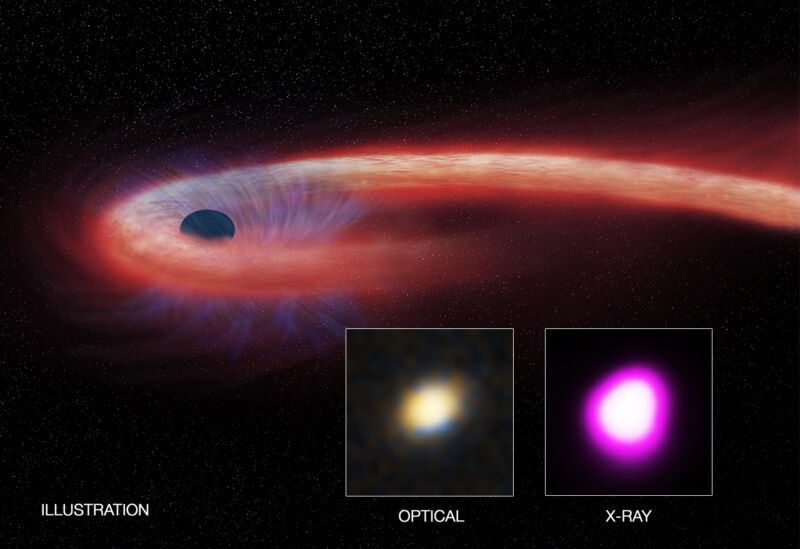
Supermassive black holes are commonly found at the center of almost every galaxy. Occasionally, a star comes too close to one of these colossal entities and undergoes a phenomenon known as a tidal disruption event. During this event, the immense gravitational force of the black hole tears the star apart, resulting in a tremendous release of radiation. This occurrence has been observed multiple times.
However, the exact reason behind the burst of radiation, which is the focus of “it,” remains somewhat elusive. Stars typically emit radiation through fusion, but the spaghettification of the star caused by the tidal disruption event effectively terminates the fusion reactions. While black holes do brighten when consuming matter, this process does not resemble the sudden surge of radiation observed during a tidal disruption event.
Interestingly, the production of radiation in this context still holds uncertainties. Various competing theories exist, yet determining the most fitting explanation based on the available data has proven challenging. Nonetheless, scientists have made use of an updated software package to simulate a tidal disruption event, and their enhanced model aligns quite well with our observations.
Spaghettification simulation
As previously mentioned, there is still uncertainty surrounding the radiation source in tidal disruption events. While these events are known to be significant and catastrophic, the specifics of the radiation emitted, such as the dominant wavelengths and the rate at which its intensity fluctuates, can provide valuable insights into the underlying physics.
Ideally, software should serve as a link between the physics of tidal disruptions and our observations of the resulting radiation. By simulating a realistic disruption and accurately representing the physics involved, the software should generate a radiation burst that closely aligns with our observations of these events. Unfortunately, the current software has not met our expectations. In order to manage computational complexity, we have had to take numerous shortcuts, which have raised doubts about the realism of our simulations.
However, a recent study conducted by Elad Steinberg and Nicholas Stone from The Hebrew University introduces a new approach. They utilized a software package called RICH, which specializes in tracking the movement of fluids, known as hydrodynamics. Although the remnants of a star are not fluid in the conventional sense we are familiar with on Earth, their behavior is primarily governed by fluid mechanics. The latest update to RICH incorporates improved modeling of radiation emission and absorption by the materials within the fluid, making it a more suitable tool for simulating tidal disruptions.
Despite the advancements, the researchers still had to make certain compromises to ensure the computational feasibility of their simulations. The gravity model employed in the simulation is not fully relativistic and is only approximated in the immediate vicinity of the black hole. However, this approximation significantly accelerated the computations, enabling the researchers to track the star’s remnants from spaghettification to the peak of the event’s radiation output, spanning a period of approximately 70 days.
The results are shocking
The simulations indicate that the significant occurrences occur at the pericenter, which is the point in the former star’s orbit where it comes closest to the black hole. When the disrupted gas loops around and approaches this point for the second time, not all of the gas travels at the same speed. This leads to turbulence and shock waves at the pericenter, causing the gas to slow down and emit radiation.
The slowing down of the gas at the pericenter has two effects. Firstly, as time progresses, more material accumulates at the pericenter, intensifying the shockwaves and generating more radiation. Secondly, instead of the original star’s highly elliptical orbit, the turbulence-induced energy loss brings the material exiting the pericenter into a more circular orbit.
The resulting structure resembles a tadpole, with a long, thin tail of stretched material and a denser oval structure resembling the head, encompassing the black hole. The majority of the radiation is emitted by the shockwaves occurring on the top of the head.
Steinberg and Stone argue that this transition to a more circular orbit is a self-reinforcing process. As a small portion of the material slows down, the turbulence increases, further decelerating more material, which in turn can slow down additional incoming material. Alongside releasing orbital energy through radiation, approximately 3 percent of the incoming material is ejected from the vicinity of the black hole, contributing to the energy balance.
However, as more material accumulates in slower orbits, the incoming streams have significantly less mass than the material they collide with. Consequently, the shockwaves weaken and more radiation is absorbed by the surrounding material. This leads to the fading of the radiation from the tidal disruption event.
Overall, the researchers’ simulation does not precisely match any of the individual tidal disruption events observed.
The simulations accurately capture the essential aspects of the actual events, indicating that they generate an idealized representation of the event while the local conditions at the black hole shape our observation of it. Fortunately, the viewing angle appears to have minimal impact in these simulations, alleviating one concern. Achieving a strong correlation between the simulations and real-world data is a promising beginning. However, considering the proximity to a supermassive black hole, the researchers aim to further enhance these simulations by incorporating a software version that effectively accounts for relativistic effects.
Do not forget to share your opinion with us to provide you with the best posts !
0 Comments