This Australian experiment is looking for an illusive particle that could be able to provide some light on the nature of dark matter.
Australian scientists are making strides towards solving one of the greatest mysteries of the universe: the nature of invisible “dark matter”.
A scientist and scientific communicator based in Perth and Melbourne is Ben McAllister. He is employed at Swinburne University and the University of Western Australia.
Australian researchers are making progress in understanding the nature of invisible “dark matter,” one of the universe’s greatest mysteries.
A common contender among theories that attempt to explain dark matter, the axion was recently searched for by the ORGAN Experiment, Australia’s first significant dark matter detector.
The quest for axions has been made more focused because to ORGAN, which has set additional restrictions on the qualities that they could possess. But before we go too far forward…
Let’s start with a story
The fundamental particles that would eventually come to be known as you, the planet, and the galaxy were all gathered into one extremely hot, dense location some 14 billion years ago.
Then, after the Big Bang, everything broke apart. The particles came together to form atoms, which later congregated to form stars, which erupted and produced a wide variety of exotic stuff.
Earth appeared after a few billion years, and ultimately it was teeming with little creatures we now name humans. Cool tale, huh? It turns out that this isn’t even half of the tale.
The “regular stuff” that makes up people, planets, stars, and galaxies is the same. However, we are aware that just one-sixth of the universe’s matter is composed of ordinary stuff.
What we refer to as “dark matter” makes up the remainder. You can nearly infer everything about it from its name. It contains mass and doesn’t emit light, therefore we term it “dark” (so we call it “matter”).
If it’s invisible, how do we know it’s there?
We repeatedly discover while observing how objects move in space that our findings cannot be explained by taking into account merely what we can see.
A good example are galaxies that are spinning. The gravitational attraction of visible stuff alone cannot account for the rotational velocities of the majority of galaxies.
Therefore, these galaxies must include dark matter, which provides more gravity and enables them to spin more quickly without having pieces fling off into space. Our hypothesis is that dark matter actually binds galaxies together.
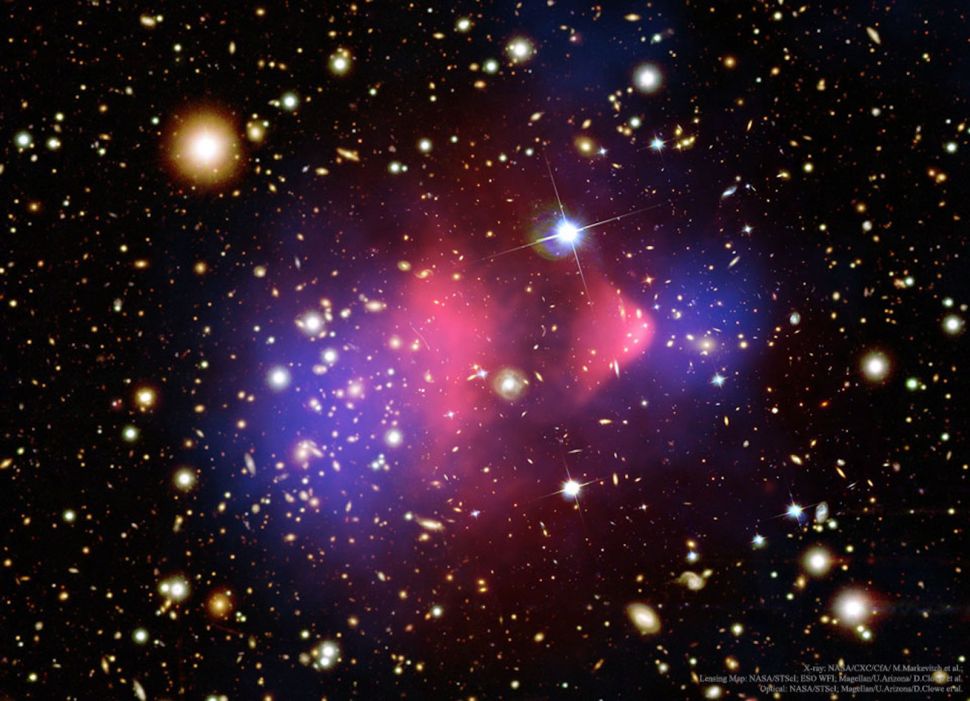
As a result of the dark matter dragging on everything we can see, there must be a tremendous amount of dark matter in the cosmos. Like a cosmic ghost, it is also going through you. You just cannot sense it.
How could we detect it?
Many physicists think that hypothetical axon particles might make up dark matter. Axions were first put up as a component of a solution to the “strong CP problem,” another significant issue in particle physics (which we could write a whole article about).
Anyway, once the axion was proposed, researchers discovered that, under some circumstances, the particle may potentially make up dark matter. This is due to the fact that axions are predicted to have mass and very weak interactions with ordinary matter, which are two requirements for dark matter.
What is the best way to look for axions then?
We can construct detectors right here on Earth because it is believed that dark matter surrounds us. Fortunately, the theory that predicts axions also predicts that, given the correct circumstances, axions may transform into photons, or particles of light.
This is good news because humans are excellent photon detectors. And ORGAN does this function precisely. It creates the ideal circumstances for the conversion of axions into photons and searches for faint photon signals, or tiny flashes of light caused by dark matter passing through the detector.
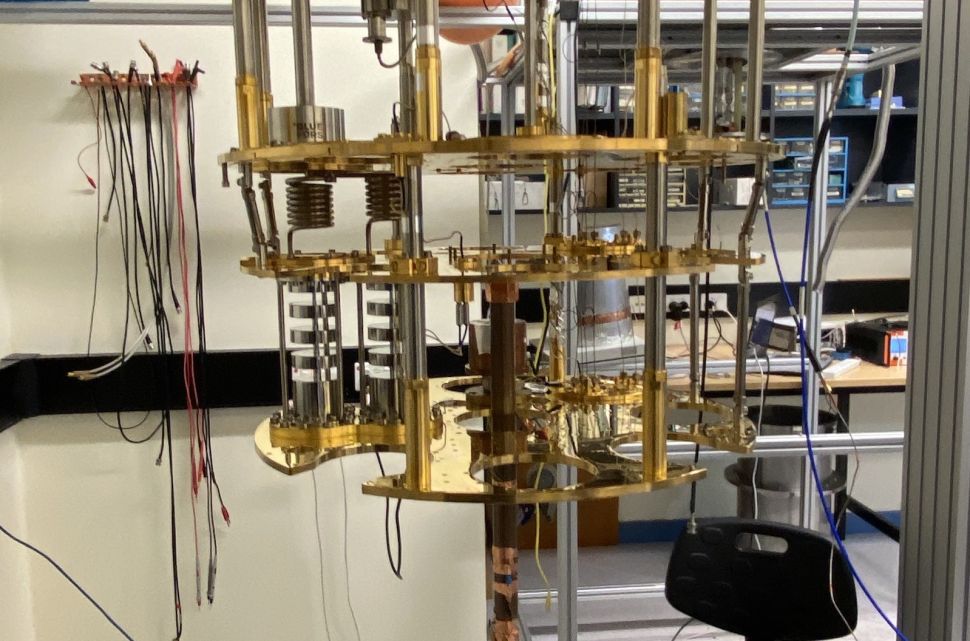
Shining a light on dark matter
A strong magnetic field is thought to cause an axion to change into a photon. We create this magnetic field in a conventional haloscope using a sizable electromagnet known as a “superconducting solenoid.”
One or more metal hollow chambers are positioned inside the magnetic field with the intention of trapping photons there and causing them to bounce around, making them simpler to detect.
There is one snag, though. Small, sporadic flashes of light are continually emitted by everything that has a temperature (which is why thermal imaging cameras work). The sporadic emissions, or “noise,” makes it more challenging to find the elusive dark matter signals.
We’ve put our resonator in a “dilution refrigerator” to get around this. With the experiment being cooled to cryogenic temperatures—roughly 273°C—the noise is much reduced.
We can “listen” for feeble photons produced during dark matter conversion more effectively the colder the experiment is.
Targeting mass regions
Axions of a particular mass will change into photons of a particular wavelength or hue. However, since the mass of axions is unknown, investigations must concentrate their search in areas where dark matter is thought to be more likely to exist.
If no dark matter signal is detected, either the experiment’s sensitivity is insufficient to distinguish the signal from background noise, or there is no dark matter in the axion mass area.
When this occurs, we establish a “exclusion limit,” which simply means that we were unable to detect any dark matter within this mass range with the sensitivity we had. This instructs the other dark matter researchers to focus their efforts elsewhere.
The most sensitive experiment in the intended frequency range is ORGAN. In its most recent run, it found no signs of dark matter. This study has established a significant exclusion boundary for the potential properties of axions .
The quest for axions is in its first stages and will go for several years. The following experiment, which will be more sensitive and aim for a new, uncharted mass range, is now being prepared.
But why does dark matter matter?
Well, for one thing, we know from past experience that spending money on fundamental physics results in the creation of significant technology. For instance, our comprehension of quantum physics is a prerequisite for all modern computers.
If we had not been interested in things that at the time seemed to be weird physical occurrences that were beyond our comprehension, we would never have found electricity or radio waves. The same applies to dark matter.
Think about all that humanity has done by comprehending just one-sixth of the universe’s matter, then picture what we could accomplish if we could unlock the remainder.
This article is republished from The Conversationunder a Creative Commons license. Read the original article.
Do not forget to share your opinion with us to provide you with the best posts !
0 Comments