Observing Quantum Avalanches in a Many-Body Localized System through Experimentation
Strongly correlated systems refer to collections of particles that exhibit intense interactions, where the behavior of each particle is intricately linked to the actions of all other particles in the system. In states significantly removed from equilibrium, these systems can manifest intriguing and unexpected physical phenomena, such as many-body localization. Many-body localization occurs when a system comprising interacting particles resists reaching thermal equilibrium, even at elevated temperatures. In such systems, particles persist in a non-equilibrium state for extended durations, even in the presence of substantial energy flow.
Theoretical predictions propose that the instability of the many-body localized phase arises from minute thermal inclusions within the strongly interacting system, acting as a bath. These inclusions trigger the delocalization of the entire system through a mechanism known as avalanche propagation.
A recent study conducted by researchers in Markus Greiner’s group at Harvard University delves into this captivating yet experimentally elusive phenomenon. Their work, highlighted in Nature Physics, marks the first experimental observation of the initiation of quantum avalanches in a many-body localized system.
The significance of the research is emphasized by Julian Léonard, one of the study’s researchers, who shared with Phys.org, “Whether particles remain localized in a disordered potential, or whether they spread out, is a long-standing question that has occupied physicists for many decades.
This question is important because in materials, localization is linked to electronic transport, hence understanding the conditions when particles localize will tell us why certain materials are insulators or conductors.”
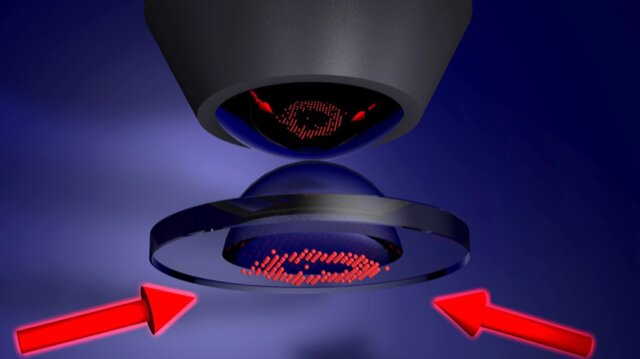
The phenomenon of particle localization is rooted in quantum mechanics, relying on the wave nature of electrons and the process of entanglement, wherein particles, such as electrons, become intricately correlated through quantum mechanical means. Gaining a more profound comprehension of localization stands as a crucial objective within the physics community, carrying the potential to significantly impact both research and the development of technology.
Firstly, the exploration of perfect localization presents an intriguing research avenue due to its defiance of thermodynamics, a widely recognized and firmly established theory in physics. Secondly, a system featuring perfectly localized particles could serve as a notable subject of study, as it has the capability to retain quantum information for extended durations. Consequently, unraveling the underlying mechanisms of perfect localization has the potential to propel advancements in quantum technology, particularly in the domain of quantum memories.
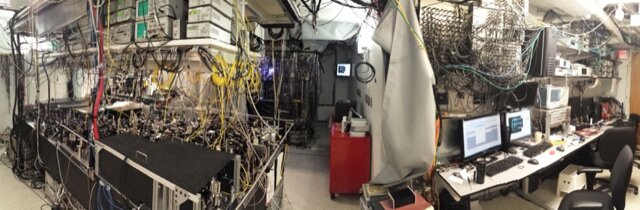
“These quantum memories are indispensable for quantum computing and communication protocols,” remarked Léonard. “Several research groups, including ours, had previously observed the localization of interacting particles, with a widespread consensus that this localization should endure indefinitely. However, recent debates have centered around the robustness of localization, particularly under slightly weaker disorder in certain parts of the system. Could this weakened disorder suffice to dismantle localization?
” The primary aim of Léonard and his colleagues in their recent study was to meticulously scrutinize localization and its resilience. Previous theoretical calculations posited an intricate and captivating scenario wherein localization could be disrupted. In particular, theorists anticipated that, under specific conditions, particles in a region with mild disorder could swiftly migrate towards a strongly disordered segment of the system, leading to delocalization. Termed a quantum avalanche, this phenomenon resembles a wave of localized particles surging towards the delocalized region, rapidly accelerating and causing the entire system to lose localization, akin to an avalanche.
“To realize such a system experimentally in the lab posed a challenge for us,” noted Léonard. “To address this, we introduced cold atoms into a potential created with precisely shaped laser beams. One part of the potential exhibited disorder, while the other remained disorder-free. Subsequently, we observed the interaction between these two regions over time and measured the extent to which particles spread. In the realm of cold atoms, this can be meticulously achieved by observation with an optical microscope.” Remarkably, Léonard and his colleagues observed that initially, particles in the disordered section of their system localized. However, over time, particles from the non-disordered region began to rapidly spread into the disordered area, aligning with theoretical predictions. These observations signify the successful exploration of the initiation of a quantum avalanche in an experimental setting for the first time. Intriguingly, this suggests that localization may not be as robust as previously believed, and its endurance may be limited.
These compelling findings are likely to stimulate further experiments aimed at probing quantum avalanches and assessing the stability of localization in strongly interacting many-body systems. “Our experiments mark the discovery of quantum avalanches, but they are just the beginning of exploring their properties,” Léonard emphasized. “Many questions remain open, particularly under which conditions these avalanches occur, how frequently they manifest, and whether there are means to impede their propagation. These factors will ultimately determine whether localization is inherently unstable or only under specific conditions. We are presently working on establishing systems with more atoms to delve into these questions in greater detail.”
This article is republished from PhysORG under a Creative Commons license. Read the original article.
Do not forget to share your opinion with us to provide you with the best posts !
0 Comments