New Phase of Matter Opens a Portal to an Additional Time Dimension
The ancient Incas utilized a device comprised of many strings called a quipu to store tax and census records, encoding the information in knots. After several hundred years, scientists are close to creating a much more advanced modern version. Their knots are produced by patterns of laser pulses that essentially establish a second dimension of time. Their “quipu” is a new phase of matter produced within a quantum computer.
This isn’t quite as difficult to understand as it first seems. The new phase belongs to a family of several other phases known as topological phases, which were initially discovered in the 1980s. These materials exhibit order not as a result of the regular spacing of atoms in a crystal, but rather as a result of the dynamic movements and interactions between their components. Applying unique combinations of electromagnetic fields and laser pulses to bring order or “symmetry” to the movements and states of a substance’s atoms results in the creation of a new topological phase, or new “phase of matter.” Induced repeating movements are one example of how such symmetries might occur in time rather than space.
Time symmetries can be hard to observe directly, but they can be mathematically explained by viewing the real-world material as a projection from a fictitious higher-dimensional space, much like a two-dimensional hologram is a projection of a three-dimensional item. It is possible to identify the symmetries of this newly generated phase, which appears as a strand of ions (electrically charged atoms), by seeing it as a substance existing in a multidimensional world with two temporal dimensions.
As a team member who was present for the experiments at the Flatiron Institute in New York City, Philipp Dumitrescu says, “It is very exciting to see this unusual phase of matter realized in an actual experiment, especially because the mathematical description is based on a theoretical ‘extra’ time dimension.” On July 20, a publication outlining the research appeared in Nature.
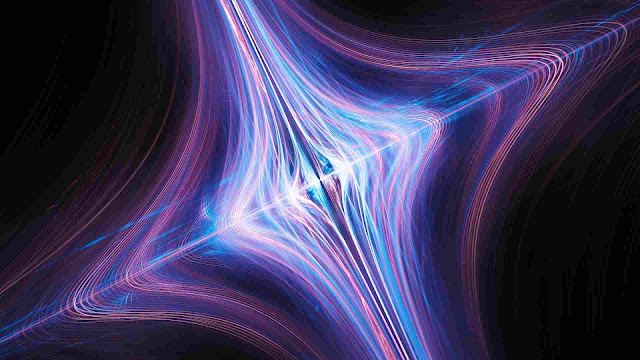
Though it may sound exciting to open a gateway to an additional temporal dimension, this was not the physicists’ first goal. According to Andrew Potter, a quantum physicist at the University of British Columbia and a research co-author, “We were very much inspired to discover what new sorts of phases might be developed.” The research members didn’t understand it may aid in error-proofing data being processed by quantum computers until they visualized their suggested new phase.
The predicted power of quantum computers comes from the ability of quantum bits, or qubits, to store values of either 0 or 1 or both simultaneously (think of Schrödinger’s cat, which can be both dead and alive). Standard classical computers encode information as strings of bits—0’s or 1’s—whereas quantum computers are predicted to be more powerful. The majority of quantum computers store information in the state of each qubit, for example, in the spin of a particle, which can point up or down and represent a 0 or 1 or both at once. However, any disturbance, like a stray magnetic field, might cause chaos on a perfectly constructed system by flipping spins arbitrarily or even wiping out quantum effects altogether, stopping operations.
Potter compares this weakness to writing a message on the ground using bits of string, each one organized into the shape of a different letter. You could read it quite well up until a light breeze blows a letter away, he claims. Potter’s team turned to topological phases to make the more error-proof quantum material. Information is not locally encoded in the state of each qubit in a quantum computer that makes use of topology, but rather is globally woven throughout the material. According to Potter, it’s similar to the Incas’ system for keeping census and other data—a knot that’s hard to untie—quipu.
Justin Bohnet, a quantum physicist at the Broomfield, Colorado-based business Quantinuum, who is also a research co-author, explains that topological phases are fascinating because they provide a mechanism to guard against material defects. This is distinct from conventional error-correction protocols, which need frequent measurements on a tiny portion of the system to determine whether mistakes are present before fixing them.
The H1 quantum processor from Quantinuum is composed of a strand of 10 qubits—10 ytterbium ions—that are precisely controlled by lasers in a vacuum chamber. A common method used by physicists to control ions is a “ion trap.” Potter, Dumitrescu, and their colleagues originally attempted to produce a topological phase that would be stable against mistakes by giving the ions, which were all arranged in one dimension, periodic kicks with repeatedly repeating laser pulses. According to Potter’s calculations, “the quantum processor would be protected against mistakes” as a result. Similar to how a constant drumbeat may keep a group of dancers in time, this is possible.
The researchers tested each time the program was run on Quantinuum’s processor to see whether the quantum state of all the qubits matched their theoretical predictions to determine if they were correct. Laughing, Potter adds, “It didn’t work at all. utterly nonsensical information was being released. Every time, the system’s performance declined within 1.5 seconds of growing mistakes. The group eventually came to the conclusion that only adding one time symmetry was insufficient. In reality, Potter says, the periodic laser pulses were magnifying minuscule glitches in the system, making small disturbances much worse, rather than shielding the qubits from being impacted by external bumps and noise.
He and his colleagues went back to the drawing board so that they could finally come up with an idea: if they could create a pattern of pulses that was somehow itself ordered (as opposed to random) but did not repeat in a regular pattern, they might be able to produce a more resilient topological phase. They predicted that such a “quasi-periodic” pattern could be able to create many symmetries in the ytterbium qubits of the processor without causing any undesirable amplifications. They settled on the well-known mathematical Fibonacci sequence, in which each successive number equals the sum of the two preceding ones. (Thus, a normal periodic laser pulse sequence such as A, B, A, B… may alternate between two frequencies from two lasers.
The system, according to Potter, may be easily thought of as “two lasers pulsating with two separate frequencies” that ensure the pulses never temporally overlap, even if these patterns really originated from a somewhat complicated arrangement of two collections of different laser pulses. The theoretical portion of the team envisaged these two independent collections of beats along two distinct time lines for the purposes of its computations; each collection is essentially throbbing in its own time dimension. On the surface of a torus, one may trace these two temporal dimensions. The way each of the two time lines repeatedly wraps around the torus “at a peculiar angle that never repeats on itself,” according to Potter, reveals the quasi-periodic structure of the time lines.
The processor of Quantinuum was actually shielded when the researchers put the new software into use with the quasi-periodic sequence throughout the entire test duration of 5.5 seconds. It may not seem like much in terms of seconds, but the change is noticeable, according to Bohnet. It is evident that the demonstration is effective.
Chetan Nayak, a quantum computing specialist at Microsoft Station Q at the University of California, Santa Barbara who was not involved in the project, concurs that it is “quite fascinating.” He points out that while two-dimensional spatial systems are more difficult and expensive to construct than one-dimensional ones, they often provide superior error prevention. The team’s invention of the effective second time dimension skirts around this restriction. According to him, “their one-dimensional system behaves in certain ways like a higher-dimensional system without incurring the expense of creating a two-dimensional system.” You may enjoy the best of both worlds by “having your cake and eating it, too.”
The tests are “elegant” and “fascinating,” according to Samuli Autti, a quantum physicist at Lancaster University in England who was also not on the team. He is especially impressed by the fact that they involve “dynamics,” or the laser pulses and other manipulations that stabilize the system and move its individual qubits. The majority of prior attempts to topologically enhance quantum computers focused on less active control techniques, making them more static and rigid. As a result, topological protection for dynamics, according to Autti, “is a key technological priority.”
Although it is a bit of a mouthful, the term the researchers gave to their new topological phase of matter—emergent dynamical symmetry-protected topological phase, or EDSPT—recognizes its potential for transformation. Potter acknowledges that “it’d be wonderful to think of a catchier name.”
Another unanticipated benefit of the study was the discovery that the quantum computer was more error-prone than thought, as seen by the initial unsuccessful test using the periodic pulse sequence. Nayak remarks, “This was a nice opportunity to stretch and evaluate how good Quantinuum’s CPU is.”
Source:ScientificAmerican
Do not forget to share your opinion with us to provide you with the best posts !
0 Comments