Light-Driven Modulation of a Single-Molecule Electron Emitter
Researchers at University of Tokyo, JTS PRESTO, Ludwig Maximilians Universität and Kindai University recently demonstrated the modulation of an electron source by applying laser light to a single fullerene molecule. Their study, featured in Physical Review Letters, could pave the way for the development of better performing computers and microscopic imaging devices.
“By irradiating a sharp metallic needle with femtosecond pulses, we had previously demonstrated optical control of electron emission sites on a scale of approximately 10 nm,” Hirofumi Yanagisawa, one of the researchers who carried out the study, told Phys.org. “The optical control was achieved using plasmonic effects, but it was technically difficult to miniaturize such an electron source using the same principle. We were seeking a way to miniaturize the electron source and we hit upon the idea of using a single molecule and its molecular orbitals.”
Yanagisawa and his colleagues set out to realize their idea experimentally using electrons emitted from molecules on a sharp metallic needle. However, they were well-aware of the difficulties they would encounter, due to unresolved difficulties associated with the use of electron emissions from molecule-covered needles.
“For one, it was not clear whether electron emissions originate from single molecules or not, and beyond that, the interpretation of the emission patterns was not clear,” Yanagisawa explained. “Although there were mysteries that we had to clarify, we thought that light-induced electron emissions from molecule-covered needles would be a new phenomenon anyway, if we could observe this, and that the phenomena would give us answers to those intractable questions.”
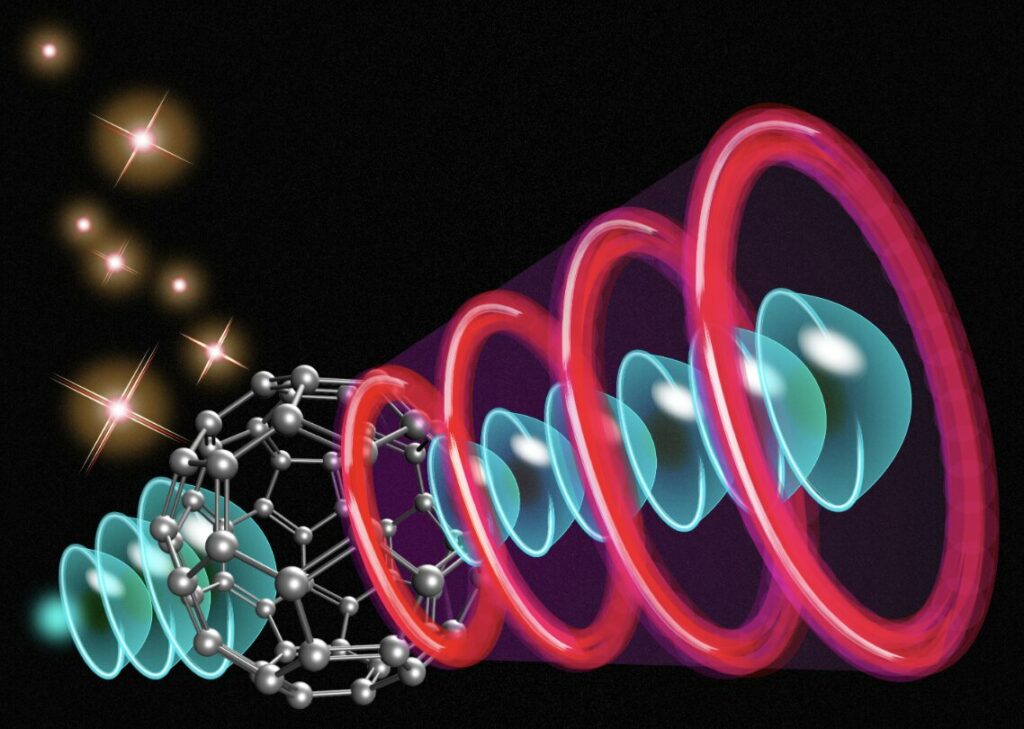
One year after they started conducting their experiments, the researchers successfully observed light-induced changes in electron emission patterns. Understanding the physics underpinning this observed phenomenon, required an additional four years of research.
To miniaturize a site-selective electron source via the so-called plasmonic effect, researchers first need to change the shape of an electron emitter at an atomic scale, which is a highly technical and challenging task. Instead of changing the shape of the emitter, therefore, Yanagisawa and his colleagues tried to change the electronic structure (i.e., molecular orbital) of electrons passing through their single-molecule emitter.
“In this case, the electronic structure in a single molecule defines a sort of aperture for incoming electron waves, where the shape of the outgoing electron waves will become the shape of the aperture,” Yanagisawa said. “For example, if the aperture is a ring shape, the outgoing electron waves also become a ring shape. The important thing is that the aperture’s shape varies with the energy of the incoming electrons in quantum mechanics.”
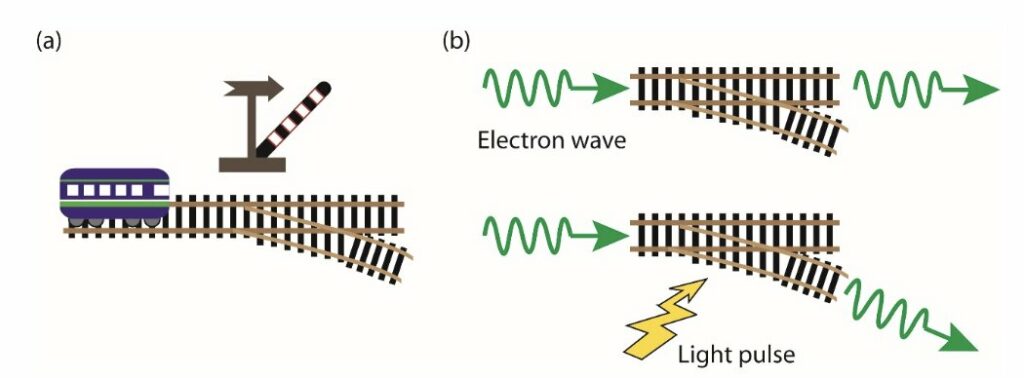
Essentially, the researchers were able to change the shape of the aperture on their emitter by exciting electrons with laser pulses and changing their energies. This in turn changed the shape of the emitted electron waves.
“We observed subnanometric modulation in electron emission sites by light,” Yanagisawa said. “Optically selecting emission sites can lead to an integration of ultrafast switches that can be from three to six orders of magnitude faster than switches in a computer.”
The technique introduced by the researchers could theoretically enable the integration of ultrafast switches into a single fullerene molecule. Yanagisawa and his colleagues also propose an integration scheme that would allow the integration of as many switches as desired without the need to increase the size of devices, which would generally be necessary.
In their next studies, they would like to further improve their ability to control electron emission using their technique, as this could facilitate the future integration of ultrafast switches into single molecules. In addition, they plan to explore the possibility of applying their method to electron microscopy technology.
In addition to informing the creation of vacuum nanoelectronics, in fact, their proposed method could be applied in the field of electron microscopy. The irradiation of solids using light can excite electrons within them, and some of these electrons can then be emitted into a vacuum; a process known as photoelectron emission.
“A photoelectron emission microscope (PEEM) can be used to observe femtosecond to attosecond electron dynamics on a nanoscale area,” Yanagisawa said. “Ultrafast electron dynamics play an important role, even at a single-molecule scale. However, the spatial resolution of a PEEM is around sub-10 nm or so, and hence, it was not possible to resolve electron dynamics in a single molecule.”
The modulation of light-induced electron emissions from a single molecule demonstrated by this team of researchers can be combined with PEEM technology. Yanagisawa and his colleagues showed that a PEEM based on their approach attains a spatial resolution of approximately 0.3 nm, while also resolving single-molecule molecular orbitals.
“In the future, we will be using our microscope to investigate ultrafast electron dynamics in a single molecule,” Yanagisawa added. “Because our PEEM uses low-energy electrons, we expect less damage to biomolecules so that we can observe a specific biomolecule without destroying it. Femtosecond electron dynamics play a crucial role even in photosynthesis, thus we would soon like to investigate the photosynthetic process at a single-molecule scale using our PEEM.”
This article is republished from PhysORG under a Creative Commons license. Read the original article.
Do not forget to share your opinion with us to provide you with the best posts !
0 Comments