Enhancing the Brightness of Quantum Dots: A Guide.
Quantum dots, which are artificial atoms, are incredibly small, measuring just a few nanometers in size. Composed of semiconductor materials, these tiny structures have the ability to emit light of a specific color or even single photons, making them crucial for advancements in quantum technologies. In recognition of their groundbreaking work in the commercial production of quantum dots, the discoverers and pioneers were honored with the Nobel Prize in Chemistry in 2023.
In recent times, quantum dots made from perovskites have garnered significant attention. Perovskites belong to a class of materials that share a similar structure with the mineral perovskite, specifically calcium titanate. The first production of quantum dots using perovskite materials was achieved by ETH Zurich in 2015.
These perovskite nanocrystals, when formed into a dispersion by mixing them with liquids, become easily processable. Additionally, their unique optical properties allow them to emit a brighter light compared to many other quantum dots. Moreover, the production cost of these perovskite quantum dots is relatively lower, making them an appealing choice for various applications, such as displays.
A team of researchers, led by Maksym Kovalenko at ETH Zurich and Empa, collaborated with counterparts in Ukraine and the U.S. to further enhance the promising properties of perovskite quantum dots. Through the utilization of chemical surface treatment methods and the exploration of quantum mechanical effects previously unseen in perovskite quantum dots, they successfully demonstrated significant improvements. The findings of their research have been recently published in two papers in the esteemed journal, Nature.
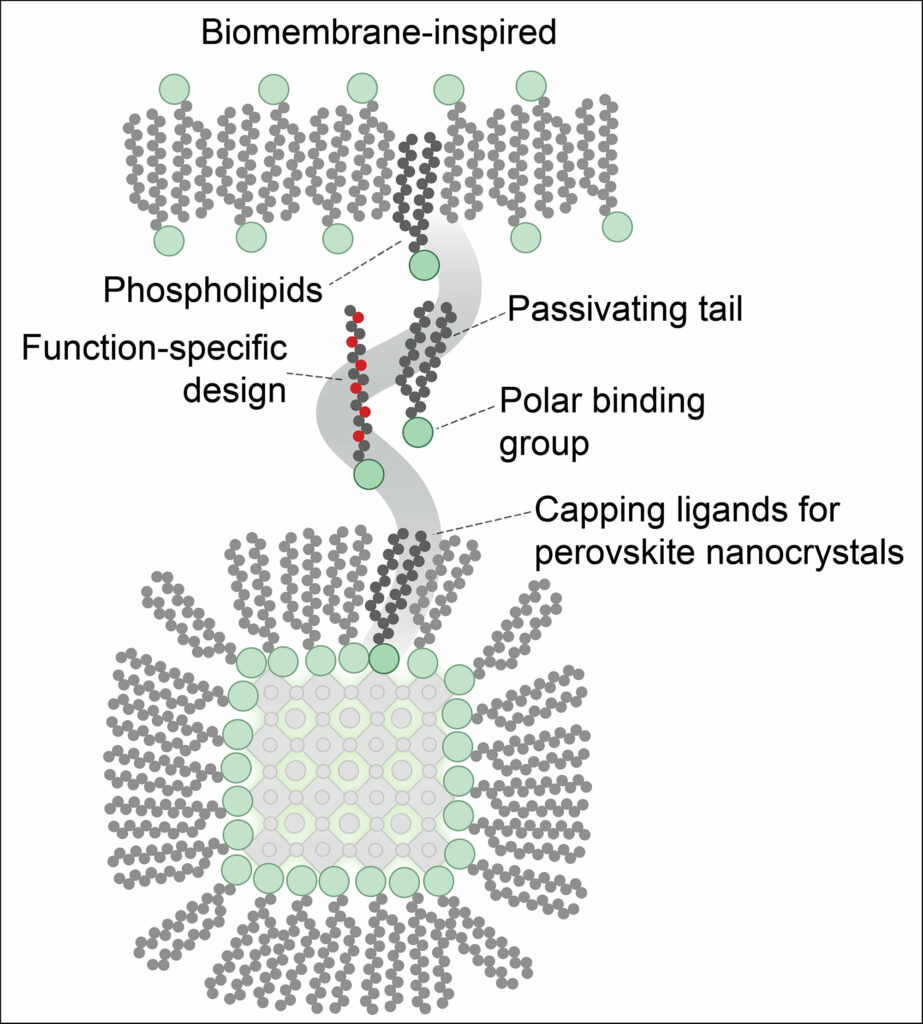
Unhappy atoms reduce brightness
Brightness serves as a crucial metric for quantum dots as it correlates with the quantity of photons emitted by the quantum dot per second. When quantum dots are stimulated, such as by higher frequency ultraviolet light, they emit photons of a specific color and frequency.
This stimulation leads to the creation of an exciton, which consists of an electron that gains more freedom to move and a hole, representing a missing electron, within the material’s energetic band structure. The excited electron can then return to a lower energy state and recombine with the hole. If the energy released during this process is converted into a photon, the quantum dot emits light.
However, this process doesn’t always occur smoothly. According to senior researcher Gabriele Raino, “unhappy” atoms lacking a neighboring atom in the crystal lattice exist on the surface of perovskite nanocrystals. These edge atoms disrupt the balance between positive and negative charge carriers within the nanocrystal, potentially causing the energy released during recombination to be converted into lattice vibrations instead of being emitted as light. Consequently, the quantum dot experiences intermittent illumination, commonly referred to as “blinking.”
Protective coating made of phospholipids
In order to prevent this occurrence, Kovalenko and his team have devised customized molecules called phospholipids. These phospholipids bear a striking resemblance to the liposomes that encapsulate the mRNA vaccine for the coronavirus, ensuring its stability in the bloodstream until it reaches the cells, as Kovalenko clarifies.
One notable distinction is that the researchers have optimized their molecules to allow the polar portion of the molecule, which is sensitive to electricity, to attach itself to the surface of the perovskite quantum dots. This ensures that the “unhappy” atoms receive a charge partner.
Furthermore, the nonpolar section of the phospholipid that extends outward enables the transformation of quantum dots into a dispersion within non-aqueous solutions like organic solvents. The lipid coating on the surface of the perovskite nanocrystals is also crucial for their structural stability, as emphasized by Kovalenko: “This surface treatment is absolutely essential for any future applications involving the quantum dots.”
Thus far, Kovalenko and his team have successfully demonstrated this treatment on quantum dots composed of lead halide perovskites, but it can be easily adapted for other metal halide quantum dots as well.
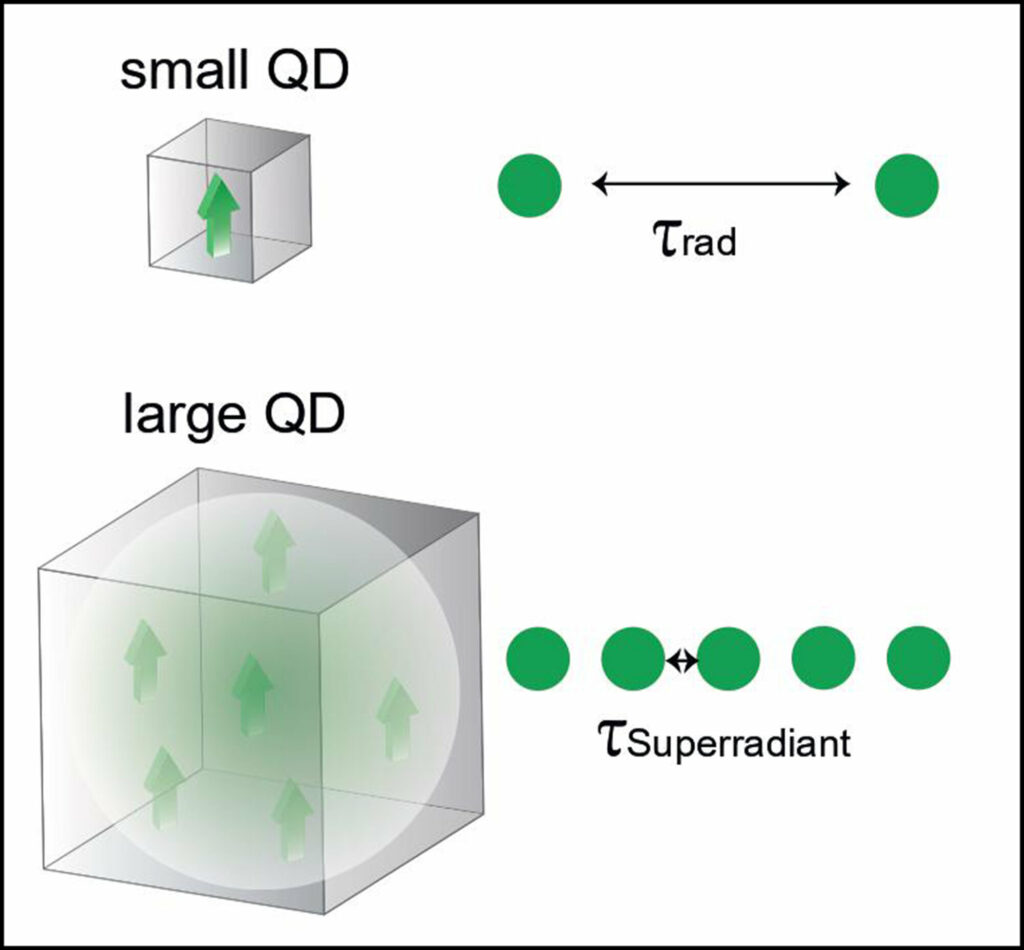
Even brighter thanks to superradiance
By utilizing the lipid surface, the researchers were able to significantly reduce the occurrence of blinking in the quantum dots. As a result, a photon was emitted in 95% of electron-hole recombination events. However, in order to further enhance the brightness of the quantum dot, the speed of recombination itself needed to be increased, which necessitates the principles of quantum mechanics.
When an excited state, such as an exciton, undergoes decay, it is due to the interaction between a dipole (consisting of positive and negative charges displacing with respect to each other) and the electromagnetic field of the vacuum. The rate of decay is directly proportional to the size of the dipole. One approach to creating a larger dipole involves coherently coupling multiple smaller dipoles together. This can be likened to pendulum clocks that are mechanically linked and synchronize their ticking after a certain period of time.
Through experimental evidence, the researchers demonstrated that coherent coupling also functions in perovskite quantum dots. In this case, there is only a single exciton dipole that, through quantum mechanical effects, spreads throughout the entire volume of the quantum dot, effectively generating multiple replicas of itself. The larger the quantum dot, the greater the number of replicas that can be produced. These replicas can induce a phenomenon called superradiance, which accelerates the recombination of the exciton.
Consequently, the quantum dot becomes ready to accept a new exciton at a faster rate, resulting in the emission of more photons per second and thereby increasing its brightness. It is important to note that the quantum dot continues to emit single photons rapidly (rather than multiple photons simultaneously), making it well-suited for applications in quantum technologies.
Kovalenko highlights that the enhanced perovskite quantum dots have a broader scope beyond light production and displays. These dots possess potential as light-activated catalysts in organic chemistry, among other less apparent domains. Kovalenko is actively investigating these applications, along with various others, under the NCCR Catalysis program.
This article is republished from PhysORG under a Creative Commons license. Read the original article.
Do not forget to share your opinion with us to provide you with the best posts !
0 Comments