Attoscience Reveals a Light-Matter Hybrid Phase in Graphite Resembling Superconductivity
X-ray absorption spectroscopy, a method known for its element-selective and electronic-state sensitivity, has long been a widely utilized analytical technique for studying material composition. However, until recently, this method required laborious wavelength scanning and lacked ultrafast temporal resolution for investigating electronic dynamics.
Over the past decade, the Attoscience and Ultrafast Optics group at ICFO, led by ICREA Professor Jens Biegert, has transformed attosecond soft-X-ray absorption spectroscopy into an innovative analytical tool. This advancement eliminates the need for scanning and introduces attosecond temporal resolution.
Attosecond soft-X-ray pulses, ranging from 23 attoseconds to 165 attoseconds, along with a coherent soft-X-ray bandwidth spanning from 120 to 600 eV, enable the simultaneous interrogation of a material’s entire electronic structure. The integration of real-time time resolution for detecting electronic motion and coherent bandwidth for pinpointing the occurrence of changes establishes a completely new and potent tool for solid-state physics and chemistry.
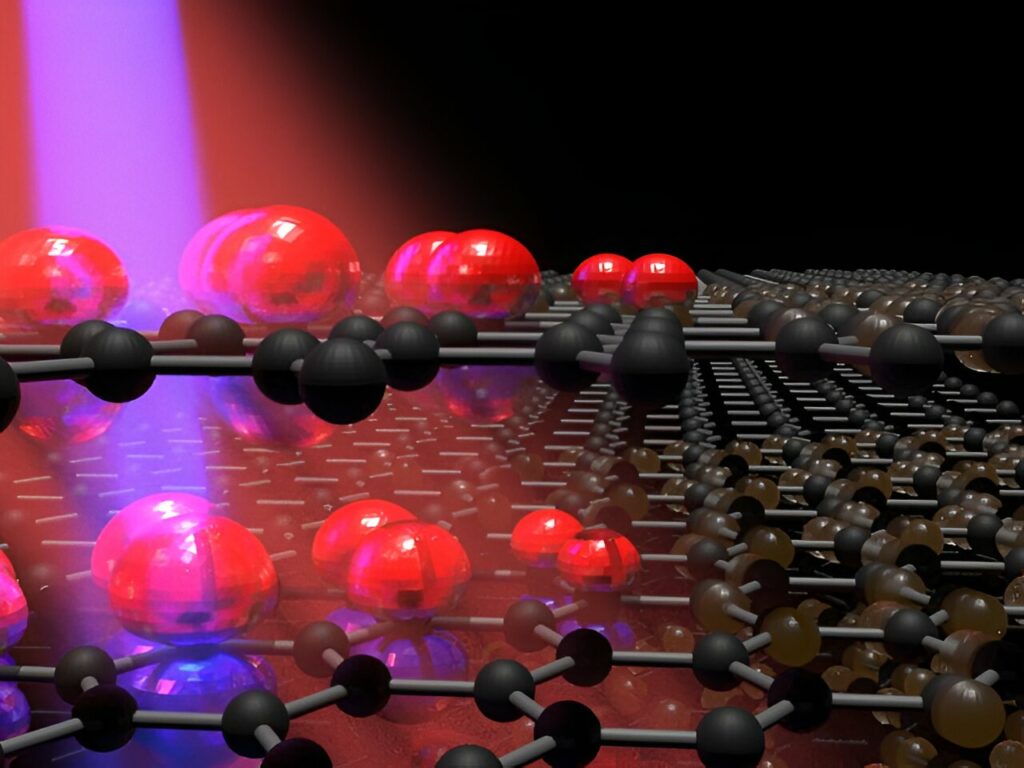
One of the fundamentally crucial processes involves the interaction between light and matter, for instance, in comprehending the mechanisms behind solar energy absorption in plants or the conversion of sunlight into electricity in solar cells. A pivotal facet of material science revolves around the potential to modify the quantum state or function of a substance through exposure to light.
Exploring the dynamics of materials at the many-body level addresses key challenges in modern physics, such as identifying the triggers for quantum phase transitions or understanding how material properties emerge from microscopic interactions. In a recent publication in Nature Communications, researchers from ICFO—Themis Sidiropoulos, Nicola Di Palo, Adam Summers, Stefano Severino, Maurizio Reduzzi, and Jens Biegert—reveal their observation of a light-induced enhancement and control of conductivity in graphite. This achievement is attributed to the manipulation of the many-body state of the material.
Attosecond pump-probe measurement of the light-matter hybrid
The researchers utilized carrier-envelope-phase-stable sub-2-cycle optical pulses at 1850 nm to induce a light-matter hybrid state. They examined the electronic dynamics using attosecond soft-X-ray pulses with a duration of 165 as at the carbon K-edge of graphite (285 eV).
The attosecond soft-X-ray absorption measurement probed the complete electronic structure of the material at attosecond-interval pump-probe delay steps. The 1850 nm pump led to a high conductivity state in the material, known as a light-matter hybrid, solely existing due to the light-matter interaction. Researchers are intrigued by such conditions as they are anticipated to yield quantum properties absent in equilibrium, switchable at optical speeds up to many THz. However, the exact manifestation of these states inside materials remains largely unclear. Unlike experimental approaches such as twistronics and twisted bilayer graphene, where physical manipulation of samples reveals electronic property changes, Sidiropoulos clarified, “
Instead of manipulating the sample, we optically excite the material with a powerful light pulse, exciting the electrons into high energy states and observe how these relax within the material, not only individually but as a whole system, watching the interaction between these charge carriers and the lattice itself.”
The study, led by Themis Sidiropoulos, emphasizes the need for coherent probing, attosecond time resolution, and synchronization between pump and probe for these investigations facilitated by attosecond science. Observing the relaxation of electrons in graphite after the intense light pulse, the researchers noted an increase in the material’s optical conductivity, exhibiting characteristics reminiscent of a superconductivity phase. Coherent phonons, observed in a prior publication, played a crucial role. The strong phonons efficiently transported energy away from the crystal without damaging it, creating artificial superconductivity signatures as electrons rode the wave. These findings hold promise for applications in photonic integrated circuits and optical computing, where light manipulates electrons, offering innovative ways to explore and control correlated phases of matter in real-time—a pivotal aspect for modern technologies, as highlighted by Biegert.
This article is republished from PhysORG under a Creative Commons license. Read the original article.
Do not forget to share your opinion with us to provide you with the best posts !
0 Comments