A novel approach to fabricate and control superconductors at elevated temperatures.
Physicists have been fascinated by superconductors for many years. However, these materials, enabling the flawless and lossless transmission of electrons, typically manifest this quantum-mechanical phenomenon only at extremely low temperatures—just a few degrees above absolute zero—which renders their practical application challenging.
Under the leadership of Harvard Professor of Physics and Applied Physics, Philip Kim, a research team has successfully showcased a fresh approach to create and control a well-explored category of higher-temperature superconductors known as cuprates. This breakthrough opens the door to the engineering of novel and unconventional forms of superconductivity in materials that were previously considered unattainable.
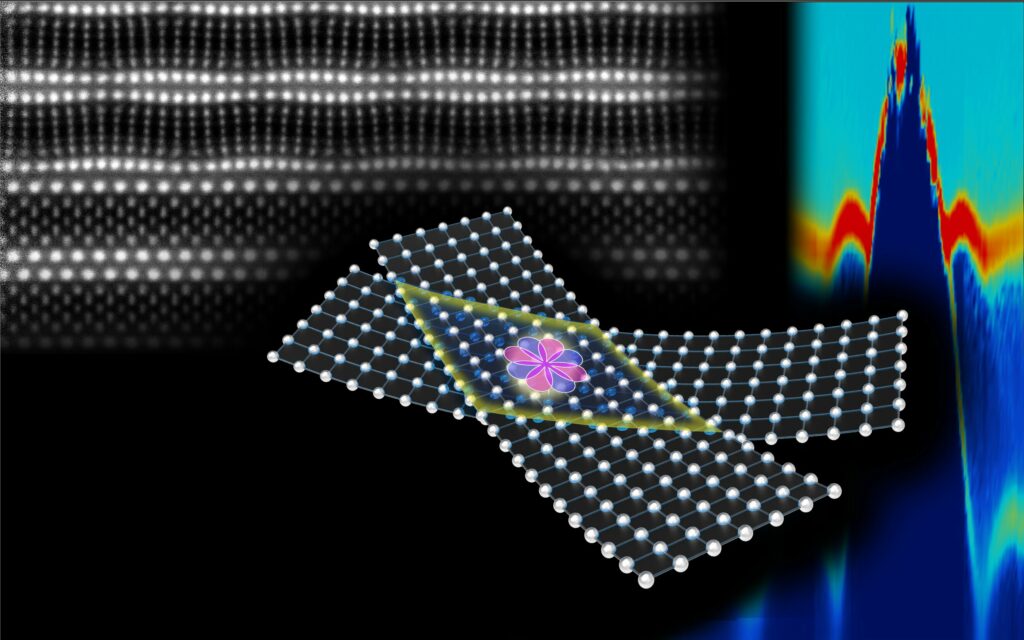
The research endeavors were spearheaded by S. Y. Frank Zhao, a former student of the Griffin Graduate School of Arts and Sciences and currently a postdoctoral researcher at MIT. Employing an air-free, cryogenic crystal manipulation technique within ultrapure argon, Zhao meticulously engineered a pristine interface between two exceptionally thin layers of the cuprate bismuth strontium calcium copper oxide, affectionately known as BSCCO or “bisco.”
Characterized as a “high-temperature” superconductor, BSCCO initiates superconductivity at approximately -288 Fahrenheit (-177 C)—a temperature considered very cold in practical terms but remarkably high within the realm of superconductors, which typically require cooling to around -400 Fahrenheit (-240 C). Zhao initiated the process by dividing the BSCCO into two layers, each measuring a mere one-thousandth the width of a human hair. Subsequently, at -130 F (-90 C), he stacked the two layers at a 45-degree twist, resembling an ice cream sandwich with slightly skewed wafers, while maintaining superconductivity at the delicate interface.
The team’s findings revealed that the maximum supercurrent passing through the interface without resistance varied depending on the direction of the current. Significantly, the team showcased electronic control over the interfacial quantum state by reversing this polarity. This ability to control the quantum state was instrumental in creating a switchable, high-temperature superconducting diode—an illustration of fundamental physics that holds the potential for integration into computing technologies, such as quantum bits. “This marks the beginning of exploring topological phases, featuring quantum states protected from imperfections,” noted Zhao.
Collaborating with Zhao were colleagues Marcel Franz at the University of British Columbia and Jed Pixley at Rutgers University. These collaborators had previously conducted theoretical calculations predicting the behavior of the cuprate superconductor at various twist angles. Additionally, reconciling the experimental observations required new theoretical developments, undertaken by Pavel A. Volkov at the University of Connecticut.
This article is republished from PhysORG under a Creative Commons license. Read the original article.
Do not forget to share your opinion with us to provide you with the best posts !
0 Comments